Snag Dynamics in Western Oregon and Washington
Janet L. OhmannJuly 26, 2002
INTRODUCTION
To achieve desired amounts and characteristics of snags and down wood, managers require analytical tools for projecting changes in dead wood over time, and for comparing those changes to management objectives such as providing dead wood for wildlife and ecosystem processes. The following information on rates of snag recruitment, decay, and fall across forests of western Oregon and Washington may be useful in planning for future levels of dead wood. Eventually the information will be incorporated directly into dead wood dynamics models. In the meantime, existing dead wood dynamics models still can be used to predict changes in dead wood levels and comparing to target levels.The summaries presented here are based on remeasurement data from permanent Forest Inventory and Analysis (FIA) plots. The plots sample several DecAID vegetation conditions on the west side of the crest of the Cascade Mountains in Washington and Oregon (“westside”), but are predominantly from the Westside Lowland Conifer-Hardwood wildlife habitat type. Similar tree remeasurement data are available for eastern Washington and Oregon, but have not yet been compiled. Repeat measurements on permanent plots to determine tree death and fall are very reliable, whereas estimates of change in snag height and decay class are less accurate and are not presented here. Also, because repeatable measurements of down wood along transects are problematic, data on down wood dynamics at the individual log level are unlikely to be provided by regional forest inventory plots. The information presented here therefore focuses on live tree mortality, snag creation, and snag fall.
Cartography is by Matt Gregory. Bruce Marcot provided the bibliographies on dead wood dynamics.
METHODS
Rates of snag recruitment, decay, and fall were summarized from repeat measurement data for FIA plots in western Oregon and western Washington (Table 1, Figure 1). Each plot and tree was measured twice over a 10-year period. On the initial visit to each plot, all live and standing dead trees were tallied and species, height, diameter at breast height (DBH), and decay class were recorded. At the second visit, trees previously tallied as snags were noted as still standing, fallen, or harvested. If snags were still standing, their current DBH, height, and decay class were recorded. Trees that died since the first measurement (mortality) were noted as still standing (recruited snags) or fallen, and cause of death was recorded.Because snag dynamics are strongly influenced by logging and forest management activities, data were analyzed separately for disturbed and undisturbed plots. ‘Disturbed’ plots were those where any kind of tree cutting or silvicultural treatment (clearcut, partial harvest, precommercial thin, commercial thin, incidental harvest) was recorded for the 10-year remeasurement period. Disturbances that occurred prior to the remeasurement period are not accounted for in the analysis. Snag recruitment (fall rate of mortality trees) and snag fall also are summarized by State and by tree size class.
KEY FINDINGS
Snag Recruitment
C Rates of mortality tree recruitment as snags varied by cause of death (Table 2). Trees killed by insects, animals, and suppression were most likely to remain standing as snags over the 10-year remeasurement period. Not surprisingly, trees killed by weather (including windthrow) and root disease were most likely to fall down soon after death.C Nearly half of all mortality trees, dying from all causes combined, fell within 10 years of death and were not recruited as snags (Table 2).
Rates of Snag Fall and Harvest
C In undisturbed stands, 30% of all snags >25.4 cm (10.0 in) DBH fell down over the 10-year period (Table 3).C Snag fall rates in undisturbed stands were substantially lower for the largest snags: most (93%) snags >100 cm (39 in) DBH remained standing over the remeasurement period (Table 3). Snags in the two smaller DBH classes fell at about the same rate (30% and 33%).
C Snag retention was strongly impacted by harvest activities. Only 15% of snags >25 cm (10 in) DBH remained standing after disturbance (Table 4). Most snags (62%) were cut down and either left on site or removed. A smaller percentage (16%) fell down naturally. Smaller snags were more likely to be cut down than larger snags.
C In undisturbed stands, western redcedar and ‘other conifer’ (mostly cedar) snags stood the longest (Table 5.). Hardwoods, Sitka spruce, and true firs fell at the greatest rates.
MANAGEMENT IMPLICATIONS
These findings have several implications for planning for desired future conditions of snags. The high fall rate (almost half) of recent mortality trees needs to be considered when planning for future recruitment of snags and down wood. Trees that fall soon after death provide snag habitat only for very short periods of time or not at all, but do contribute down wood habitat. In fact, these trees are a desirable source of down wood as they will often begin as mostly undecayed wood and, if left on the forest floor, will proceed through the entire wood decay cycle with its associated ecological organisms and processes that are beneficial to soil conditions and site productivity (see section on Ecosystem Processes Related to Wood Decay). Because many existing snag dynamics models assume that all mortality trees are recruited as snags, a major implication of these findings is that these models will substantially overestimate future snag abundance and underestimate amounts of down wood.The cause of tree death also needs to be considered in planning for snags and down wood. Trees killed by insects, animals, suppression, and diseases other than root disease are most likely to remain standing as snags. The quality of snag habitat also varies with mortality agent and should be considered as well -- see insect and disease discussions for more information.
Because snag retention is so strongly affected by harvest activities, dead wood should be planned for separately for disturbed and undisturbed stands. Although 62% of snags on disturbed plots in our study were cut down, it’s likely that more snags could be retained in harvest units than our findings indicate, by applying creative approaches to snag placement that also address safety and operational concerns (see Rose et al. 2001, p. 605 and Neitro et al. 1985). Our findings suggest that snag size (DBH) and species should be considered when identifying particular snags to retain in harvest units. The larger the snag diameter, the more likely it is to survive harvest operations and remain standing in future years. Species of cedar can be expected to stand the longest (although cedar tends to have low overall wildlife value , whereas hardwoods, Sitka spruce, and true firs will be shortest-lived (but often have higher wildlife value (see the Ancillary Data section in summary narratives)). Again, both the quality and the longevity of snag habitat needs to be considered (see the Considerations for Stand Dynamics section in summary narratives).
Forest Vegetation Simulators and Dead Wood Dynamics Models
Dead wood dynamics models can be used to project the recruitment, fall, and decay of snags and down wood on a site to determine amounts and characteristics of dead wood over time (see Rose et al. 2001, p. 605). Dead wood dynamics models can guide efforts at green tree retention and snag and down wood creation. Estimates of tree mortality can be obtained from forest simulation models such as the Forest Vegetation Simulator (FVS), Organon , or Zelig, and entered into the dead wood dynamics models as recruited snags and down wood. Existing dead wood dynamics models do not yet incorporate all of the recently available information on tree dynamics from remeasurement of permanent plots such as presented in this paper. In the future, forest simulators and dead wood dynamics models will be updated and improved to include the best available information. Future improvements to DecAID will include more in-depth guidance on using dead wood dynamics models in planning for future dead wood to meet management objectives for wildlife, biodiversity, and ecosystem processes.In the meantime, several existing dead wood dynamics models are available for use, which are described in Rose et al. 2001, p. 605: the Coarse Wood Dynamics Model (CWDM) (Mellen and Ager), the Snag Recruitment Simulator (SRS) (Marcot), and the Snag Dynamics Projection Model (SDPM) (McComb and Ohmann). In addition, the Forest Vegetation Simulator (FVS) now has a Fire and Fuels Extension (FFE) that tracks and simulates decay and fall of standing dead trees, and decay of down wood (i.e., ‘surface fuels’). The variants for Oregon and Washington have recently (January 2006) been updated to include new information on snag dynamics.
More information on dead wood dynamics can be found in the published literature. Bibliographies on the dynamics of snags and down wood, and on dynamics of wood decay, are appended to the end of this paper.
Table 1. Forest Inventory and Analysis plot data in western Oregon and Washington.
![]() | Western Oregon | Western Washington |
Number of plots | 338 | 669 |
% of plots undisturbed* over remeasurement period | 68 | 72 |
Inventory dates | Mid-1980s, mid-1990s | Late 1970s, late 1980s |
Number of mortality trees | -- | 499 |
Number of remeasured snags | 1,128 | 2,076 |
Table 2. Fate of mortality trees of all species and >25.4 cm (10.0 in) DBH by cause of death over a 10-year period, western Washington.
Cause of death | Percent still standing | Percent fell down |
Insects | 96 | 4 |
Root disease | 52 | 48 |
Other rots | 63 | 37 |
Animals | 100 | 0 |
Weather | 28 | 72 |
Suppression | 79 | 21 |
Other / unknown | 61 | 39 |
All causes | 56 | 44 |
Table 3. Fate of remeasured snags over a 10-year period by diameter class (DBH) in undisturbed* stands, western Oregon and Washington.
Snag fate | <25.4-50.0 cm (10-20 in) | 50.1-100.0 cm (20-39 in) | >100.0 cm (39 in) | All sizes |
Percent still standing | 61 | 59 | 93 | 62 |
Percent fallen | 30 | 33 | 4 | 30 |
Percent shrank to <25.4 cm (10.0 in) DBH or <2 m (7 ft) tall | 9 | 8 | 3 | 8 |
Table 4. Fate of remeasured snags by snag size over a 10-year period by diameter class (DBH) in disturbed* stands, western Oregon and Washington.
Snag fate | <25.4-50.0 cm (10-20 in) | 50.1-100.0 cm (20-39 in) | >100.0 cm (39 in) | All sizes |
Percent still standing | 8 | 15 | 42 | 15 |
Percent fallen | 17 | 17 | 3 | 16 |
Percent shrank to <25.4 cm (10.0 in) DBH or <2 m(7 ft) tall | 3 | 9 | 8 | 7 |
Percent cut down | 72 | 59 | 47 | 62 |
Table 5. Fate of remeasured snags over a 10-year period by species in undisturbed* stands in western Oregon and Washington.
Snag fate | Douglas-fir | Western hemlock | Western redcedar | Sitka spruce | True fir | Other conifers** | Hard- woods |
Percent still standing | 70 | 67 | 76 | 30 | 37 | 78 | 29 |
Percent fallen | 23 | 20 | 12 | 67 | 40 | 22 | 65 |
Percent shrank to <25.4 cm (10.0 in) DBH or <2 m (7 ft) tall | 7 | 13 | 12 | 3 | 23 | 0 | 5 |
** Incense cedar, Alaska yellow-cedar, Port-Orford cedar, redwood, Pacific yew, and mountain hemlock.
Figure 1. Plot locations and topography.
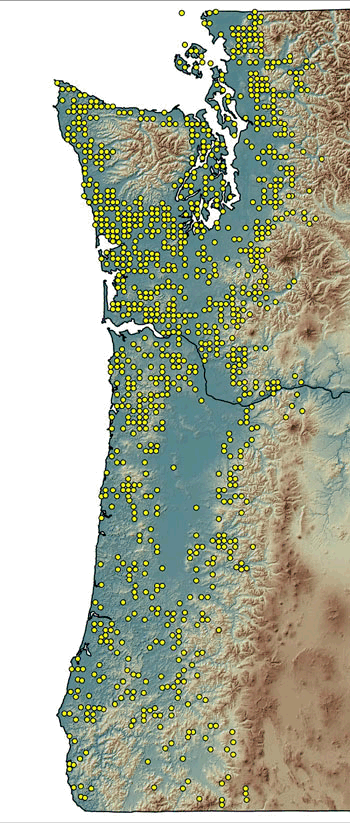
Bibliography on Dynamics of Snags and Down Wood
Current as of July 26, 2002Aubry, K. B., B. L. Biswell, J. P. Hayes, and B. G. Marcot. In press. Use of live trees and snags by mammals and their effects on ecosystem function. Pp. xxx-xxx in: C. Zabel and R. Anthony, ed. Mammal community dynamics in coniferous forests: management and conservation in the new millennium. Cambridge University Press, Cambridge MA.
Cline, S. P., A. B. Berg, and H. M. Wright. 1980. Snag characteristics and dynamics in Douglas-fir forests, western Oregon. Journal of Wildlife Management 44:773-786.
Edman, M., and B. G. Jonsson. 2001. Spatial pattern of downed logs and wood-decaying fungi in an old-growth Picea abies forest. J. Vegetation Science 12(5):609-620.
Everett, R., J. Lehmkuhl, R. Schellhaas, P. Ohlson, D. Keenum, H. Riesterer, and D. Spurbeck. 1999. Snag dynamics in a chronosequence of 26 wildfires on the east slope of the Cascade Range in Washington state, USA. International Journal of Wildland Fire 9(4):223-234.
Fridman, J., and M. Walheim. 2000. Amount, structure, and dynamics of dead wood on managed forestland in Sweden. Forest Ecology and Management 131(1-3):23-36.
Gonor, J. J., J. R. Sedell, and P. A. Benner. 1988. What we know about large trees in estuaries, in the sea, and on coastal beaches. Pp. 83-113 in: C. Maser, R. F. Tarrant, J. M. Trappe, and J. F. Franklin, ed. From the forest to the sea: a story of fallen trees. Gen. Tech. Rpt. PNW- GTR-229. USDA Forest Service, Portland OR.
Graham, R. L. L. 1981. Biomass dynamics of dead Douglas-fir and western hemlock boles in mid- elevation forests of the Cascade Range. Ph.D. Dissertation, Department of Forestry. Oregon State University, Corvallis, Oregon. 152 pp.
Grier, C. C. 1978. A Tsuga heterophylla - Picea sitchensis ecosystem of coastal Oregon: decomposition and nutrient balances of fallen logs. Can. J. For. Res. 8:198-206.
Gutzwiller, K. J., and S. H. Anderson. 1987. Short-term dynamics of cavity-nesting bird communities in disjunct floodplain habitats. The Condor 89:710-720.
Harmon, M. E., and C. Hua. 1991. Coarse woody debris dynamics in two old-growth ecosystems. BioScience 41:604-610.
Huggard, D. J. 1999. Static life-table analysis of fall rates of subalpine fir snags. Ecological Applications 9(3):1009-1016.
Keen, F. P. 1955. The rate of natural falling of beetle-killed ponderosa pine snags. J. Forestry 53:720-723.
Keim, R. F., A. E. Skaugset, and D. S. Bateman. 2000. Dynamics of coarse woody debris placed in three Oregon streams. Forest Science 46(1):13-22.
Kruys, N., B. G. Jonsson, and G. Stahl. 2002. A stage-based matrix model for decay-class dynamics of woody debris. Ecological Applications 12(3):773-781.
Maser, C., and J. M. Trappe. 1984. The seen and unseen world of the fallen tree. USDA Forest Service General Technical Report PNW-164. Pac. NW. For. Rng. Expt. Stn., Portland, OR. 56 pp.
Maser, C., and M. J. Trappe. 1984. The fallen tree--a source of diversity. Pp. 335-339 in: New forests for a changing world. Proc. Soc. Amer. For. Natl. Conf., 1983 Oct 16-20, Portland OR. Soc. Amer. For., Bethesda MD.
Maser, C., R. F. Tarrant, J. M. Trappe, and J. F. Franklin. 1988. From the forest to the sea: a story of fallen trees. USDA Forest Service Pacific Northwest Forest and Range Experiment Station General Technical Report PNW-GTR-229. USDA Forest Service, Portland OR.
Mellen, K., and A. Ager. Submitted. A coarse wood dynamics model for the western Cascades. (pp. xxx-xxx) In: P. J. Shea and W. Laudenslayer (Ed.). Proceedings of a conference on The Ecology and Management of Dead Wood in Western Forests, Reno, Nevada. USDA Forest Service, Pacific Southwest Research Station General Technical Report PSW-XXX.
Morrison, M. L., and M. G. Raphael. 1993. Modeling the dynamics of snags. Ecol. Applic. 3(2):322-330.
Parks, C. G., D. A. Conklin, L. Bednar, and H. Maffei. 1999. Woodpecker use and fall rates of snags created by killing ponderosa pine infected with dwarf mistletoe. RP-515. USDA Forest Service, Portland OR. 11 pp.
Raphael, M. G., and M. L. Morrison. 1987. Decay and dynamics of snags in the Sierra Nevada, California. Forest Science 33:774-783.
Schmid, J. M., S. A. Mata, and W. F. McCambridge. 1985. Natural falling of beetle-killed ponderosa pine. USDA Forest Service Research Note RM-454, Rocky Mountain Experiment Station. USDA Forest Service,
Sedell, J. R., P. A. Bisson, F. J. Swanson, and S. V. Gregory. 1988. What we know about large trees that fall into streams and rivers. Pp. 47-82 in: C. Maser, R. F. Tarrant, J. M. Trappe, and J. F. Franklin, ed. From the forest to the sea: a story of fallen trees. Gen. Tech. Rpt. PNW- GTR-229. USDA Forest Service, Portland OR.
Sinton, D. S., J. A. Jones, J. L. Ohmann, and F. J. Swanson. 2000. Windthrow disturbance, forest composition, and structure in the Bull Run Basin, Oregon. Ecology 81(9):2557-2566.
Spies, T. A., and S. P. Cline. 1988. Coarse woody debris in forests and plantations of coastal Oregon. Pp. 5-23 in: C. Maser, R. F. Tarrant, J. M. Trappe, and J. F. Franklin, ed. From the forest to the sea: a story of fallen trees. Gen. Tech. Rpt. PNW- GTR-229. USDA Forest Service, Portland OR.
Tinker, D. B., and D. H. Knight. 2000. Coarse woody debris following fire and logging in Wyoming lodgepole pine forests. Ecosystems 3:472-483.
Welty, J. J., T. Beechie, K. Sullivan, D. M. Hyink, R. E. Bilby, C. Andrus, and G. Pess. 2002. Riparian aquatic interaction simulator (RAIS): a model of riparian forest dynamics for the generation of large woody debris and shade. Forest Ecology and Management 162(2-3):299-318.
Wilhere, G. F. 2003. Simulations of snag dynamics in an industrial Douglas-fir forest. Forest Ecology and Management 174(1-3):521-539.
Bibliography on Dynamics of Wood Decay
Current as of July 26, 2002Amaranthus, M. P., D. S. Parrish, and D. A. Perry. 1989. Decaying logs as moisture reservoirs after drought and wildfire. (pp. 191-194) In: E. Alexander (Ed.). Stewardship of soil, air and water resources. Wathershed 89. R10-MB-77. USDA Forest Service, Region 10, Juneau, Alaska.
Boyce, J. S. 1932. Decay and other losses in Douglas Fir in western Oregon and Washington. USDA Forest Service Tech. Bull. No. 286. USDA Forest Service, Washington DC. 60 pp.
Buchanan, T. S., and G. H. Englerth. 1940. Decay and other volume losses in wind-thrown timber on the Olympic Peninsula, Washington. USDA Forest Service Tech. Bull. No. 733. USDA Forest Service, Wasington DC. 30 pp.
Conner, R. N., D. C. Rudolph, D. Saenz, and R. R. Schaefer. 1994. Heartwood, sapwood, and fungal decay associated with red-cockaded woodpecker cavity trees. J. Wildl. Manage. 58(4):728-734.
Fischer, W. C., and B. R. McClelland. 1983. A cavity-nesting bird bibliography - including related titles on forest snags, fire, insects, disease, and decay. Gen. Tech. Rep. INT-140. USDA Forest Service, Ogden UT. 79 pp.
Graham, R. L., and K. Cromack. 1982. Mass, nutrient content, and decay rate of dead boles in rain forests of Olympic National Park. Can. J. For. Res. 12:511-521.
Jonsson, B. G. 2000. Availability of coarse woody debris in a boreal old-growth Picea abies forest. J. Vegetation Science 11(1):51-56.
Kamp, B. J. V. d. 1975. The distribution of microorganisms associated with decay of western redcedar. Can. J. Forest Research 5(61):61-67.
Lindenmayer, D. B., R. B. Cunningham, and D. F. Donnelly. 1997. Decay and collapse of trees with hollows in eastern Australian forests: impacts on arboreal marsupials. Ecol. Applic. 7(2):625-641.
McFee, W. W., and E. L. Stone. 1966. The persistence of decaying wood in the humus layer of northern forests. Soil Sci. Soc. Am. Proc. 30:512-516.
Muller, M. M., M. Varama, J. Heinonen, and A. M. Hallaksela. 2002. Influence of insects on the diversity of fungi in decaying spruce wood in managed and natural forests. Forest Ecology and Management 166(1-3):165-181.
Przybylowicz, P. R., B. R. Kropp, M. E. Corden, and R. D. Graham. 1987. Colonization of Douglas-fir poles by decay fungi during air-seasoning. Forest Products Journal 37(4):17-23.
Rose, C. L., B. G. Marcot, T. K. Mellen, J. L. Ohmann, K. L. Waddell, D. L. Lindley, and B. Schreiber. 2001. Decaying wood in Pacific Northwest forests: concepts and tools for habitat management. Pp. 580-623 in: D. H. Johnson and T. A. O'Neil, ed. Wildlife-habitat relationships in Oregon and Washington. Oregon State University Press, Corvallis OR.
Silvester, W. B., P. Sollins, T. Verhoeven, and S. P. Cline. 1982. Nitrogen fixation and acetylene reduction in decaying conifer boles: effects of incuabation time, aeration, and moisture content. Can. J. Forest Research 12(3):646-652.
Sollins, P. 1982. Input and decay of coarse woody debris in coniferous stands in western Oregon and Washington. Canadian Journal For. Res. 12:18-28.
Sollins, P., S. P. Cline, T. Verhoeven, D. Sachs, and G. Spycher. 1987. Patterns of log decay in old-growth Douglas-fir forests. Canadian Journal of Forest Research 17:1585-1595.
Thomas, G. P., and R. W. Thomas. 1954. Studies in forest pathology. XIV. Decay of Douglas-fir in the coastal region of British Columbia. Can. J. Botany 32:630-653.
Wilcox, W. W. 1968. Changes in wood microstructure through progressive states of decay. USDA Forest Service Research Paper FPL 70. Forest Products Laboratory. USDA Forest Service, Madison, WI. 46 pp.
Zabel, R. A., F. F. Lombard, and A. M. Kenderes. 1980. Fungi associated with decay in treated Douglas-fir transmission poles in the northeastern United States. Forest Products Journal 30(4):51-56.